Journal Search Engine
ISSN : 2287-545X(Online)
DOI : https://doi.org/10.5656/KSAE.2012.04.0.012
보리나방살이고치벌에 대한 행동 반응으로서의 화랑곡나방 유충의 용화장소 선택
초록
Pupation Site Selection Behavior of Plodia interpunctella (Lepidoptera: Pyralidae) in Response to Its Parasitoid, Bracon hebetor(Hymenoptera: Braconidae)
Abstract
- Materials and Methods
- Insects
- Experimental arena
- Experimental procedure
- Spatial distribution of pupation site of P. interpunctella
- Effect of B. hebetor on the spatial distribution of pupation site of P. interpunctella
- Functional and numerical response of B. hebetor (parasitoid) to the density of P. interpunctella (host) larvae in relation to the spatial distribution
- Paralyzing and parasitizing behavior of B. hebetor
- Data Analysis
- Results
- Pupation site of P. interpunctella in respect to larval density with and without B. hebetor
- Effect of the spatial distribution of P. interpunctella larvae on the functional response of B. hebetor
- Effect of the spatial distribution of P. interpunctella larvae on the numerical response of B. hebetor
- Discussion
- Acknowledgement
Indianmeal moth, Plodia interpunctella (Hübner), is a cosmopolitan pest that damages stored products and processed food commodities. Traditionally, the moth has been controlled by insecticides, but the efficiency of insecticides has decreased due to development of insecticide resistance (Arthur, 1996). Among the replacements of chemical control method (Schöller et al., 1997; Arthur and Phillips, 2003), biological control of P. interpunctella, using parasitoids such as trichogrammatids and braconids, has been extensively studied (Keever et al., 1985; Brower, 1988; Grieshop et al., 2006 and 2007). Among the parasitoids evaluated, Bracon hebetor Say, a gregarious larval ectoparasitoid of stored products moths (Ohh, 1993), has been considered as an effective biological control agent of P. interpunctella. Its biology and interactions with P. interpunctella populations has been extensively studied (e.g., Benson, 1974; Schöller et al., 1997; Yu et al., 1999; Bjørnstad et al., 2001; Darwish et al., 2003; Mohandas et al., 2007).
It was discovered that B. hebetor finds hosts through chemical cues produced by the host larvae and frass (Darwish et al., 2003). Yoon et al. (2005) also suggested that the substrate for P. interpunctella could be a critical factor in determining the ability of the parasitoid to search for P. interpunctella larvae; deeper larvae position in the storage of whole rice grain compared to that of rice flour or cracked rice grains may allow these larvae to escape parasitism. Variations in the spatial distribution of P. interpunctella larvae could change the interaction between P. interpunctella and B. hebetor populations and thus influence the effectiveness of B. hebetor as a biological control of P. interpunctella (Yoon et al., 2005).
The variation in spatial distribution could be caused by the behavioral response of P. interpunctella to B. hebetor. Ives (1995) emphasized the importance of the behavioral study of hosts and parasitoids in the context of spatial heterogeneity. Although the spatial distribution pattern of P. interpunctella larva in stored products has been reported (Keever et al., 1985; Yoon et al., 2005), the relationship between moth larvae distribution and the parasitoid searching behavior in addition to the functional and numerical response of the parasitoid is still unknown.
Pupation site selection by larvae is important for holometabolous insects to protect themselves from predators or parasitoids because pupae are largely defenseless. As a result of this vulnerability, insects use various strategies to defend against predators such as adjusting pupation site (West and Hazel, 1982), selecting sites away from the conspecific colony (Lucas et al., 2000), or aggregating (Jumean et al., 2011).
In this study, the relationship between the spatial distribution pattern of larvae and pupation site of P. interpunctella, and the searching efficiency of B. hebetor were evaluated. To examine this relationship, we used laboratory populations of P.interpunctella and B. hebetor in a system where the moth larvae could select pupation sites freely, which simulated the behavior of the moth in natural storage facilities (Keever et al., 1985;Yoon et al., 2005). We first examined the spatial distribution of pupation site of P. interpunctella larvae with and without being exposed to B. hebetor, then the effect of the spatial pattern on the functional and numerical response of the parasitoid.
Materials and Methods
Insects
The stock cultures of P. interpunctella and B. hebetor have been maintained in the Laboratory of population ecology at the Korea University since 1995 (Na and Ryoo, 2000) and 1996 (Yu et al., 2003), respectively. From 2003, P. interpunctella has been reared on artificial diets that consisted of wheat bran, glycerin, yeast extract, sorbic acid and methyl-p-hydroxybenzoate (1200:600:300:3:3 in volume) (modified from Silhacek and Miller, 1972). B. hebetor has been maintained on P. interpunctella culture.
The insects obtained from the stock culture were further raised in a growth chamber at 28 ± 0.5°C with a relative humidity (RH) of 75±5% and a photoperiod of 16:8 hr (L:D) under cool-white fluorescent light between 300 and 400 lux. B.hebetor from the stock culture was reared for further generation in Petri-dishes (diameter 90 mm) provided with 10 larvae (≥ fourth instar). The resulting fourth and fifth larval instars of P.interpunctella and adult B. hebetor less than 24 hrs after emergence were used for subsequent experiments.
Experimental arena
Two different acrylic cylinder was used; a eight-layer acrylic cylinder (diameter 150 mm, height 85 mm) and a single-layer cylinder (diameter 150 mm, height 50 mm). The eight-layer cylinder was used to study the spatial distribution of the pupation sites of P. interpunctella, while the single-layer cylinder was used to test the searching behavior of B. hebetor. The eight-layer cylinder consisted of a bottom, seven small acrylic rings (each ring with diameter 150 mm, height 5 mm), a lid topped with a cotton cloth ring (height 45 mm) (Fig. 1), and filled with 660 g of brown rice. The setup for the single-layer cylinder was same except the removal of the seven rings and only 83 g of brown rice added. For both setups, the grain surface was made flat with a thin, iron plate to standardize between trials.
Fig. 1. Experimental arena: (a) eight-layer cylinder and (b)single-layer cylinder.
Experimental procedure
Spatial distribution of pupation site of P. interpunctella
To study the effects of the larval density on the selection of pupation site, different numbers of larvae (2, 4, 8, 16, 32, 64 or 128) (≥ fourth instar) were introduced into an experimental cylinder and held in an incubator (Jisico, J-IBO3, Korea) set at 75±5% RH with a saturated NaCl solution at 28 ± 0.5°C, which are the optimum conditions for P. interpunctella (Na and Ryoo, 1998, 2000). When all larvae pupated, each ring with the associated brown rice was removed one by one and the number of pupae in each layer was recorded. The experiment was replicated 30 times per host density.
Effect of B. hebetor on the spatial distribution of pupation site of P. interpunctella
After three hours when P. interpunctella larvae had settled among the layers, a pair of parasitoids (female and male < 24 hours after emergence) was introduced into eight-layer cylinder. All other procedures of this experiment were the same as those described above. The parasitoids were allowed to attack the host for 24 hours and then removed from the cylinder. The cylinder was held in an incubator until both P. interpunctella and B. hebetor larvae pupated (about five days) (Kim et al., 2000). Following the same process described above, the pupation site of the moth larvae was recorded. This experiment was repeated a minimum of 30 times per larval density.
Functional and numerical response of B. hebetor (parasitoid) to the density of P. interpunctella (host) larvae in relation to the spatial distribution
The effect of spatial distribution of the P. interpunctella larvae on the ability of the parasitoid to locate the host was tested by estimating the functional and numerical response of B.hebetor in the two systems: single-layer and eight-layer cylinder (Fig. 1). The results in the single-layer cylinder served as a control to examine the spatial distribution of the moth larvae. Different numbers of moth larvae (2, 4, 8, 16, 32, 64 and 128) (≥ fourth instars) were introduced to each cylinder. After three hours, when the larvae settled among the layers, a pair of parasitoids (female and male) was introduced and allowed to attack the larvae for 24 hours and then removed. The moth larvae that were paralyzed by the parasitoid were counted.
The larvae with parasitoid eggs were transferred to a Petri-dish (diameter 35 mm) and were held in the incubator. After five days, when all the parasitoids pupated, the number of pupae per attacked larvae was recorded. Once they emerged as adults, the parasitoids were all counted and sexed. This procedure was repeated 30 or 31 times per larval density per experimental cylinder.
Paralyzing and parasitizing behavior of B. hebetor
The paralyzing and ovipositing mechanism of B. hebetor, whether the parasitoid lays eggs on the P. interpunctella larva just after it paralyzes a larva or the parasitoid paralyzes all the larvae found in a certain time period before laying eggs, is unknown. Due to the disturbance and resulting bias in the behavior caused by direct continuous observation, we used an indirect observation method that varied the time the parasitoid spent with host larvae. Sixty four larvae of P. interpunctella (≥ fourth instar) were placed into the eight-layer cylinder with the same diet (Fig. 1a). After three hours, a pair of B. hebetor (female and male ≤ 24 hours after emergence) was introduced. The number of moth larvae paralyzed and parasitized in the cylinder was recorded after 24 and 48 hours. This procedure was replicated 20 times. The changes in the proportion of parasitized larvae to total paralyzed larvae in this period were used as an index for the behavior of B. hebetor.
Data Analysis
To estimate the functional response of B. hebetor (parasitoid) to the larval density of P. interpunctella (host), the data were fit to the Holling’s disk equation (Holling, 1959) described below:
where, Na is the number of hosts attacked, a is the successful attack rate, Nt is the initial number of hosts present, Tt is the time the hosts were exposed, Pt is the parasitoid density, and Th is the handling time of the parasitoid. The parameters of the equation were estimated using a non-linear least square regression method (Proc NLIN, SAS institute, 2005). The effects of the spatial distribution of the P. interpunctella larvae on the searching ability, number of progeny produced and the progeny sex ratio of B. hebetor were evaluated using PROC TTEST and Nested ANOVA (larval density as nest) (Proc GLM, SAS institute, 2005).
Results
Pupation site of P. interpunctella in respect to larval density with and without B. hebetor
When the P. interpunctella larvae were not exposed to B. hebetor, the proportion of the pupae found in the surface layer(< 5 mm depth) was significantly higher than the number observed in the deeper layers (> 5 mm depth) (t = 5.46-23.93; df= 29; P < 0.001). Also as the larval density increased, the proportion of pupae in the surface layer was increased from 0.78 ± 0.05 to 0.86 ± 0.01 (r = 0.22; df = 207; P < 0.01) (Fig. 2). However, in case of P. interpunctella with parasitoid, the proportion of pupae found in the deeper layers (> 5 mm in depth) was significantly higher than that in the absence of the parasitoid (F = 5.60; df = 1,411; P < 0.02) (Fig. 2). In addition, there was no relationship between the proportion of the pupae in the surface layer and the larval density (r = -0.12; df = 214; P >0.05).
Fig. 2. Pupation site of Plodia interpunctella in the presence (black bar) and absence (white bar) of Bracon hebetor in the experimental cylinder.
Effect of the spatial distribution of P. interpunctella larvae on the functional response of B. hebetor
Fig. 3 shows the functional response of B. hebetor to the density of P. interpunctella larvae in the single- and eight-layer cylinder; significantly higher numbers of the moth larvae were attacked in the single-layer cylinder relative to the eight-layer cylinder (Nested ANOVA : F = 17.56; df = 1,384; P < 0.0001). The attack rate and handling time of the response curve were calculated to be 0.77 ± 0.06 and 0.013 ± 0.001 (r2= 0.94; df =180; P < 0.0001) and 0.64 ± 0.06 and 0.015 ± 0.002 (r2= 0.73; df= 214; P < 0.0001) in the single- and eight-layer cylinder,respectively.
Fig. 3. Functional response of Bracon hebetor to different densities of Plodia interpunctella larvae in the eight-layer (black circle) and single-layer cylinder (white circle). Triangles indicate the response of the parasitoid to the number of larvae in layers deeper than 5 mm. Bars indicate standard error of the means.
Effect of the spatial distribution of P. interpunctella larvae on the numerical response of B. hebetor
In both the single- and eight-layer cylinders, the number of B. hebetor pupae per attacked larvae was negatively correlated with the number of larvae attacked, with a correlation coefficient of -0.50 (df = 343; P < 0.0001). However, the number of B. hebetor pupae per attacked larva in the eight-layer cylinder was significantly higher than that in the single-layer cylinder (F= 9.58; df = 1, 330; P < 0.05) (Fig. 4).
Fig. 4. The number of Bracon hebetor pupae produced per attacked larva with respect to the densities of Plodia interpunctella larvae in the eight-layer cylinder (black circle) and the singlelayer (white circle). Bars indicate standard error of the means.
The number of female progeny produced per female B. hebetor with respect to the density of moth larvae in the both cylinders (F=0.53; df=1, 351; P>0.40) (Fig. 5) was similar when the density of the moth increased to 32, but decreased from 3.04±0.63 to 1.05±0.18 as the density increased further from 32 to 128. The progeny sex ratio (male/total) was estimated to range from 0.37±0.06 to 0.49±0.09 and was not significantly different from 0.50 in the both cylinders (Kruskal-Wallis test:x2=3.29; df=6; P>0.75) (Fig. 6).
Fig. 5. The number of female progeny produced per Bracon hebetor female with respect to the densities of Plodia interpunctella larvae in the eight-layer cylinder (black circle) and the single-layer (white circle). Bars indicate standard error of the means.
Fig. 6. Progeny sex ratio (male/total) of Bracon hebetor with respect to the number of Plodia interpunctella larvae in the eight-layer cylinder (black circle) and the single-layer (white circle). Bars indicate standard error of the means.
The data presented in Figure 7 suggests that the parasitoid attacked and paralyzed all hosts it found before laying eggs on the paralyzed larvae. The rate of the parasitized moth larvae to the attacked larvae significantly increased in the second day(F=2.27; df=714; P<0.01).
Fig. 7. Proportion of parasitized larvae of the paralyzed Plodia interpunctella larvae with respect to the time exposed to Bracon hebetor. Bars indicate standard error of the means, and significant differences (P < 0.05) are indicated by different letters over the error bars.
Discussion
We examined a behavioral response of P. interpunctella to its parasitoid, Bracon hebetor. The pupation site selection of P.interpunctella larva differed in the presence and absence of the parasitoid. When the parasitoid was present, a deeper pupation site was selected compared to when the parasitoid was absent. This difference in pupation depth may demonstrate a trade-off between survival and fitness for P. interpunctella. It has been reported that individuals who mate earlier have higher mating success and fecundity (Huang and Subramanvam, 2003), so individuals pupating near the surface would have more time to prepare for mating (i.e. dry wings). At the high density, the concentration of pupae in the surface layer may also prove the reason why P. interpunctella, in the absence of B. hebetor, tend to pupate at shallower depths (Fig. 2). However, larvae that pupate at shallower depths have a higher risk of being parasitized (Hagstrum and Smittle, 1977; Sait et al., 1997). B.hebetor seems to search for hosts primarily on the substrate surface by host chemical cues (Strand et al., 1989; Darwish et al., 2003), but the ovipositor length of B. hebetor limits its attacking depth; Akinkurolere et al. (2009) reported that a depth of 1.0 cm in an artificial diet provided refuge for P.interpunctella to escape from parasitism because the ovipositor of Habrobracon hebetor Say could not reach these depths. Our study supports this behavioral response as a defensive behavior, as searching efficiency of B. hebetor and then the number of host attacked decreased at deeper depths (Fig. 3).
Interestingly, this potential defensive behavior of the moth larvae did not affect the number of B. hebetor progeny produced; when the number of paralyzed larva decreased, the number of B. hebetor pupae produced per paralyzed larva increased significantly. This phenomenon indicates a compromise between the number of paralyzed larva and number of B. hebetor eggs laid per larva. Yu et al. (2003) reported that the number of eggs per P. interpunctella larva increased as the larval density decreased. Although indirectly, we found that B.hebetor paralyzed all the larvae found within a certain searching period before beginning to lay eggs (Fig. 7), a similar behavior was found in Microbracon hebetor Say (Ullyett, 1945). Thus, the parasitoid seems to have some mechanism to allocate its eggs in relation to the number of larvae paralyzed, resulting in the same number of offspring produced. In Microbracon hebetor Say, parasitism consists of two distinct phases: 1) locating and paralyzing hosts, and 2) relocating the paralyzed hosts to lay eggs (Ullyett, 1945). This behavior, however, is in contrast to that reported by Benson (1973); the parasitoid was shown to wait for the host, which was paralyzed just at the moment before oviposition. These contrary results suggest that B. hebetor may change its oviposition strategy based on the number of hosts it can find within a certain searching period; at a lower number of hosts, the parasitoid concentrated its eggs on the few hosts it found, whereas at a higher number of hosts it paralyzes its host and relocates before dispersing its egg to the paralyzed hosts (Fig. 7). The progeny sex ratio of B. hebetor was not affected by host distribution and did not differ from 0.5, which was in agreement with previous studies (Yu et al., 2003).
Herein, we studied the behavioral interaction between the host, P. interpunctella larva and its parasitoid, B. hebetor. The defensive behavior of P. interpunctella larvae in response to B.hebetor could inhibit total suppression of P. interpunctella, leading to the persistence of the host-parasitoid system (Hassell, 1978). Whether this persistent interaction could be a factor of inhibiting successful biological control of P. interpunctella is not clear yet. Since P. interpunctella is known to be an r-strategist (Podoler, 1974; Nam et al., 2011), the interaction between the host and parasitoid population would lead a pattern of limit cycle and thus would negatively influence on the biological control of P. interpunctella due to periodical higher density level of the moth than desired. Na et al. (2005) and Nam et al. (2011) reported that B. hebetor can suppress P. interpunctella populations only by inundating and iterative release, and that biological control of P. interpunctella by only a single release of the parasitoid could result in failure. Our behavioral study on host-parasitoid interaction would contribute to better understanding these population dynamics.
Acknowledgement
This study was supported financially by the Korea University Research Fund granted to M.I. Ryoo.
Reference




























Vol. 40 No. 4 (2022.12)
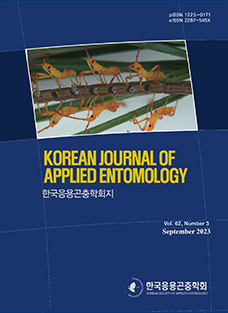
Frequency Quarterly
Doi Prefix 10.5656/KSAE
Year of Launching 1962
Publisher Korean Society of Applied Entomology



Online Submission
submission.entomology2.or.kr
KSAE
The Korean Society of Applied Entomology